Pine trees powering the future
Michelle Harnett, New Zealand Tree Grower November 2016.
We hear messages of doom and gloom nearly every day. Resources are being used faster than they can be replaced, greenhouse gas emissions are increasing, the climate is changing and rising sea levels will inundate us all. What if forestry is the solution to all these problems? A partial solution, anyway. It is time to include the concept of the bio-economy and forestry’s vital part in it and think about how developments in forest products could shape our future.
A bio-based economy
The fossil fuel economy of our everyday reality is blamed for any number of environmental and political ills. Based on finite resources, it is not sustainable in the long term. An alternative is a bio-based economy, where sustainable and renewably-grown materials are used as alternatives to oil, gas and coal to produce food, energy, chemicals and industrial products at the same time as protecting the environment and mitigating climate change.
The move to a bio-economy is already under way. The European bio-economy is worth more than €2 trillion annually and employs over 20 million people. Forestry, with its large and sustainable biomass resource, is in an excellent position to lead the way in New Zealand. However, there are a few kinks to iron out first, including how to get the most out of forestry’s resources and ensuring the sums add up. Working out how the forestry bio-economy might function is a major focus of Scion’s Manufacturing and Bioproducts division, the side of Scion dedicated to turning wood into timber, other products and energy.
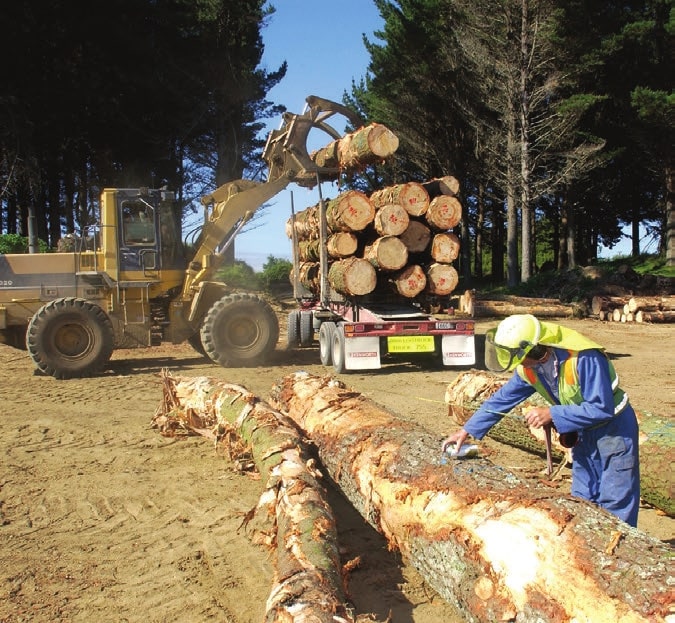
Toughening up
The radiata pine backbone of New Zealand forestry is already a bio-product. Growing sustainably, it provides income, wider ecosystem services and stores carbon as trees and timber. But being a fast growing softwood has disadvantages. Radiata lacks the toughness and appearance of hardwoods, limiting its use in some applications.
Modifying the properties of wood to improve its performance is one of Scion’s aims. It is possible to alter the function and appearance of pine by taking advantage of the chemistry of wood to change and lock its structure in place. Successfully altered, wood cell walls and the cell interiors are stable and attract little or no water, which eliminates swelling, shrinking and distortion. The altered wood is also more durable as it is often no longer recognisable as food by fungi and insects.
A simple way to do this is to heat wood in the absence of oxygen, known as thermal modification or torrefaction. The process is not new, as Vikings were known to treat wood in this way. Today, a number of commercial processes are in use around the world.
An alternative is to put out of action the reactive, water-loving chemical groups found in wood. These can be permanently modified by introducing new compounds into the wood by chemical modification The introduced compounds can form links between water-loving groups, react with them and convert them into bigger, bulkier, water-repelling groups to form polymers that fill the voids that usually contain air in dry wood.
Commercial chemically-modified wood products include Accoya, which uses acetic anhydride to plump cells up to their original green volume, and Kebony, which uses furfuryl alcohol, a polymer that links with the cell walls. Along with increased dimensional stability and durability, the wood is often darker and resembles tropical hardwoods.
Removing water
To chemically modify wood, water first has to be removed from the wet wood then the chemicals need to be introduced. Technologists have been investigating dewatering wood and further modifying it using supercritical carbon dioxide fluid extraction.
At the right combination of temperature and pressure − the supercritical point − a solvent acts as a gas and a liquid. In its supercritical state, carbon dioxide can quickly diffuse into the wood as a gas, interact with wood and sap like a liquid, then diffuse out removing the water. The supercritical process can be also be reversed to impregnate dyes, chemicals, preservatives and other functional compounds into wood. The substance to be introduced is dissolved in the supercritical fluid then deposited in in the wood.
De-watering is not a drying technology. De-watered wood looks and feels like any other piece of timber, it is heavier with a moisture content of around 40 per cent compared with 10 per cent for kiln dried timber. Scion has developed and built an automated lab-scale plant which combines de-watering and modification Recent research has focused on understanding how the process works. The novel properties of de-watered wood are also being studied and modified to achieve desired hardness, colour and durability. An early application of the patented technology produces modified radiata pine with similar physical properties and appearance to wood such as teak.
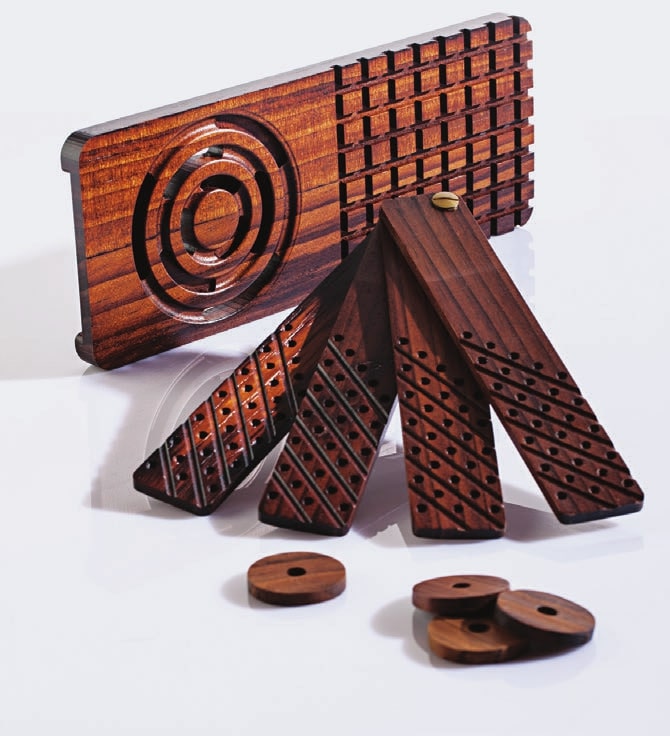
Deconstruction and reconstruction
Wood is made up of cellulose, hemicelluloses and lignin. Products such as paper are the result of separating the cellulose from the other components. Extending the idea of splitting things into their constituent parts further, the wood components can be reduced to their basic building blocks, vastly increasing the options for new products to support a bio-economy.
Nature’s glue
Lignin can be thought of as the glue that holds the cellulose fibres, and the tree, together. The basic units of lignin contain what is termed aromatic rings. These are a ring of six carbon atoms, usually shown as a hexagon with a circle inside it. Aromatic rings are a feature of solvents derived from petrochemicals such as benzene. The name aromatic comes from the smell associated with the benzene compounds when first discovered.
Benzene, along with toluene and xylene, are important industrial hydrocarbons. Around 35 million tonnes are produced from fossil fuel sources worldwide every year and used to make a range of chemicals and polymers such as polystyrene and nylon. Lignin has the potential to be used as an alternative source of aromatic rings. Thanks to the pulp and paper industry, which has been efficiently and effectively separating lignin from cellulose for decades, large supplies of lignin are readily available.
Using the inherent chemical properties of lignin was the approach taken when scientists started to develop a petrochemical-free and sustainable adhesive for wood panel products such as plywood, particleboard and MDF. The result of seven years of work is a bio-adhesive made with renewably-grown ingredients, most of which are found locally. The development process also took into account how, and where, the adhesive would be made, and how it would be used.
The patented bio-adhesive technology has been trademarked under the name Ligate. Panel boards made with Ligate have very low emissions of health-damaging chemicals and have the same performance as standard products. Panel boards made with Ligate can directly replace those currently in use, helping people live more sustainable lives.
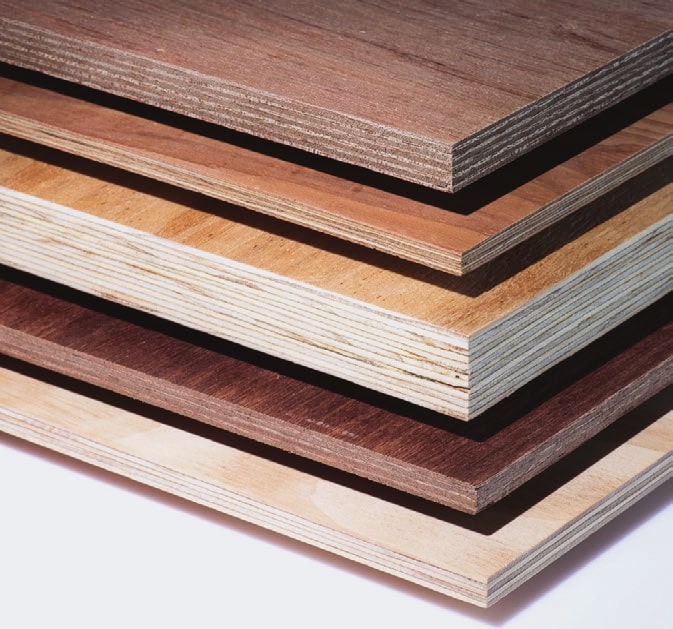
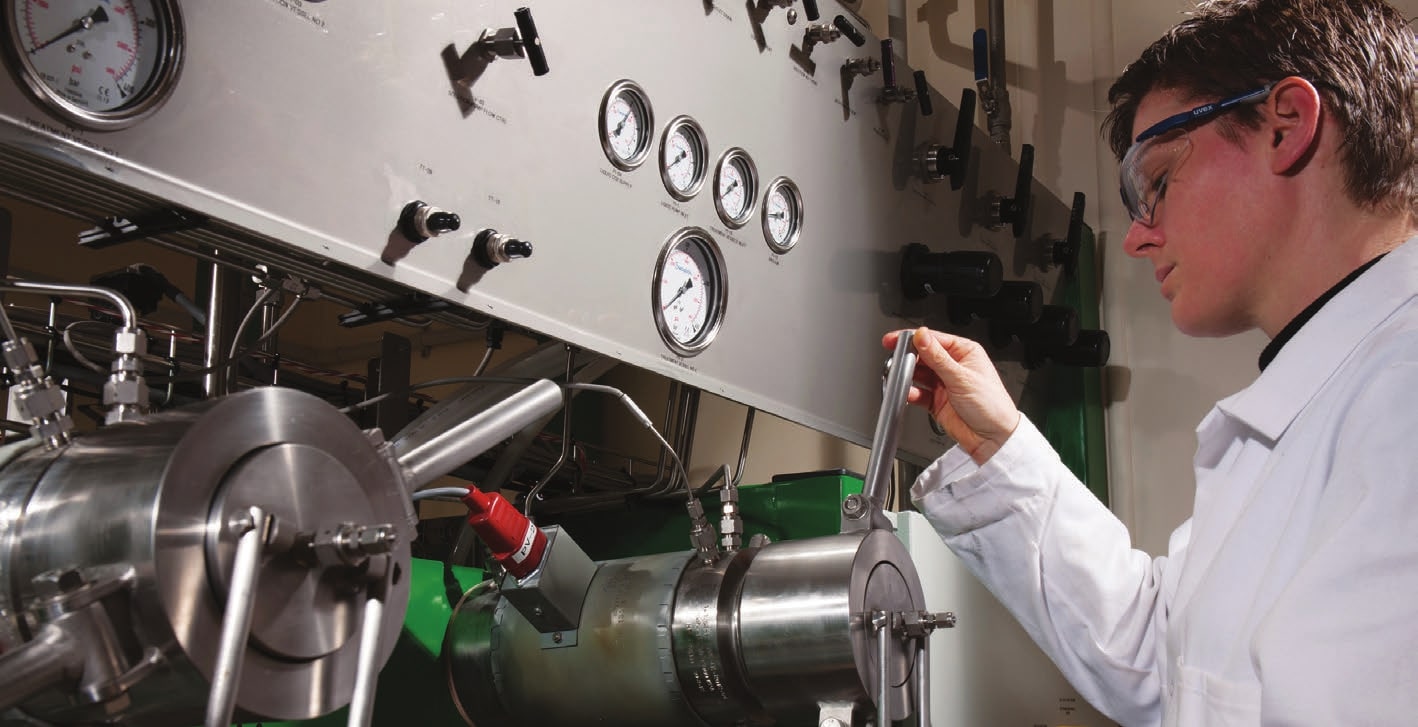
Attractive extractives
Wood also contains low levels of organic compounds, such as resins, waxes and terpenes, referred to by the general term extractives. These compounds usually play a role in protecting trees, whether it is from browsing animals, insects, fungi or microbes. Turpentine and rosin are two common examples obtained from conifers.
Extractives can be valuable. Due to their protective role, many are bioactive, with anti-microbial, antioxidant or other properties. The trick is get the extractives out efficiently and without damaging them. Traditional extraction methods include soaking plant material in water, alcohol or other solvents at different temperatures, often followed by evaporation or distillation to concentrate the active ingredients. These methods do not work well at an industrial scale.
Supercritical fluid extraction is one commercial option for removing extractives. For example, it is already used to decaffeinate coffee and extract hop compounds for beer production.
The sap removed from wood during supercritical carbon dioxide de-watering contains a range of interesting compounds, including pinenes, which are some of the most important constituents of pine resin and turpentine. Other parts of the tree and by-products of processing, such as needles or bark, can also be extracted the same way.
The path to commercial extractive products will include identifying the various extractives, separating and purifying them, followed by investigating which functions they might have. One form of pinene, for example, is known to be a bronchodilator to open up airways, anti-inflammatory and a broad spectrum antibiotic, as well as being used in industrial perfumes.
This development process has already started. Scion scientists are analysing the extracts from supercritical extraction and identifying the chemicals they contain using a chemical reference library. The work so far has produced some promising results, but before the analysts talk more widely about their findings intellectual property specialists are checking out if any of the discoveries can be patented.
Burn baby burn
Wood has been used as a source of energy for a very long time, early campfires, firing boilers to heat houses, power steam locomotives and generate electricity. In many forestry-related industries waste wood is burnt and the energy released is used somewhere in the process. Some operations are practically energy self-sufficient, burning bark, wood offcuts and sawdust to generate the steam and electricity they need.
The potential energy in wood can be extracted in ways other than burning. The process of breaking cellulose into simple sugars that can be fermented into ethanol is well known and being used worldwide. While it is currently cheaper to import sugar than to use forestry waste for ethanol production in New Zealand, wood has other rich sources of the carbon that equals energy.
Heating wood gently in the absence of oxygen changes its physical properties. Increasing the temperature of the process causes the wood to decompose into gases which can be condensed into a crude bio-diesel, and a solid carbon-rich residue called char. The process is called pyrolysis – from the Greek separation (lysis) by fire (pyro).
It is possible to use pyrolysis to produce bio-fuels. Being able to make biofuels for transportation would increase New Zealand’s fuel security and reduce greenhouse gas emissions. A small-scale fast pyrolysis plant, which can turn a kilogram of sawdust into 750 millilitres of crude bio-oil, was installed at the Rotorua campus this year.
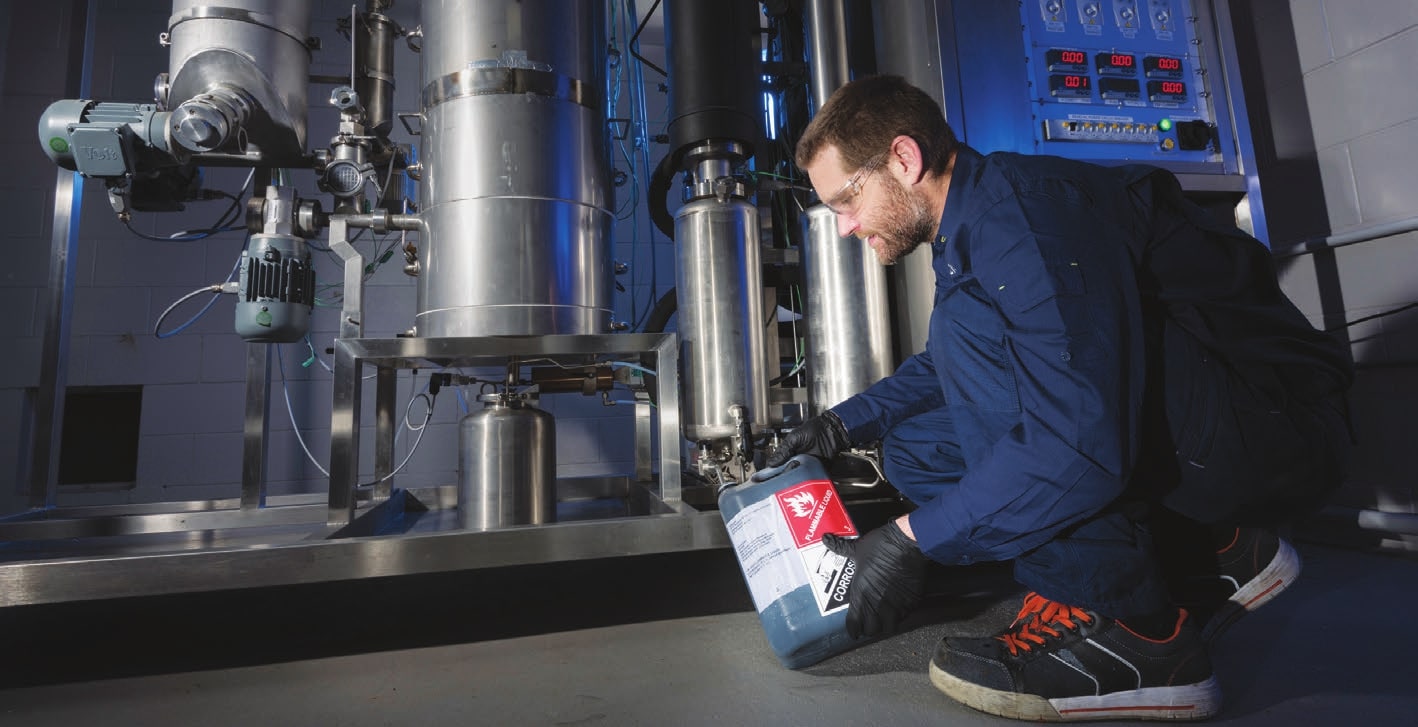
Putting the puzzle together
New technology and products emerging from laboratories are all very well, but to contribute to the bio-economy they need to be scalable commercially and make sense financially This can be particularly difficult in New Zealand, which is small in terms of population and demand but large when the distances between resources, manufacturing sites and ports are considered. We need ways to manage and integrate new technology and products within existing framework and, at the same time, invest in new infrastructure.
Building bio-refineries could be the answer. Bio-refineries convert organic materials, into power, fuel, fibre and chemicals, just as petroleum refineries produce multiple fuels and products from fossil fuel. Considering the existing infrastructure supporting forestry, sawmilling and pulp and paper manufacture, and combining them with some of the developments discussed here, it is possible to see how a bio-refinery might work.
More value from trees
Using a sawmill example, logs come in, are debarked, sawn and the timber is kiln-dried. Adding a supercritical extraction and modification unit to the operation would expand the range that could be produced on site to include high value modified wood and extractives from the de-watering process. Additionally, bark and wood waste could also undergo supercritical extraction or be subjected to pyrolysis to produce bio-fuel. Finally, any remaining material could be burned to provide additional energy for the site.
Extending the concept of combining operations, siting bio-refineries close to renewable raw materials and energy resources, and to other industries, opens up the possibility of cooperative relationships, or industrial symbiosis. When complementary industries are near one another, the by-products of one company can become the raw materials of another. Some of the advantages of this type of relationship are reduced operating costs, new business and job opportunities, especially in the regions, waste diverted from landfill and lower carbon emissions improve the sustainable credentials of New Zealand’s exports.
New technology supporting the growth of bio-refineries and industrial symbiosis will be part of the transition to a bio-based economy in which forestry, wood and biomass feature heavily. The bio-economy will realise greater value from trees, with more on-shore processing and value added to resources previously considered to be waste.
Rising log demand and farm gate log prices will encourage investment in forestry. Alongside radiata pine, alternative species or trees bred with special characteristics, such as a high terpene content, are also likely to be become more attractive to grow. Benefits for foresters and the whole country will increase with more jobs, improved regional infrastructure and generally greater prosperity. The environment will also benefit, with wiser management of natural resources, reduced dependence on fossil fuels resources and greenhouse gas emission.
Michelle Harnett is the Senior Communications Adviser for Scion.
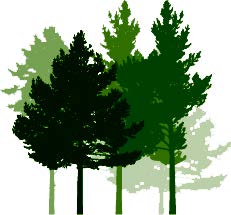